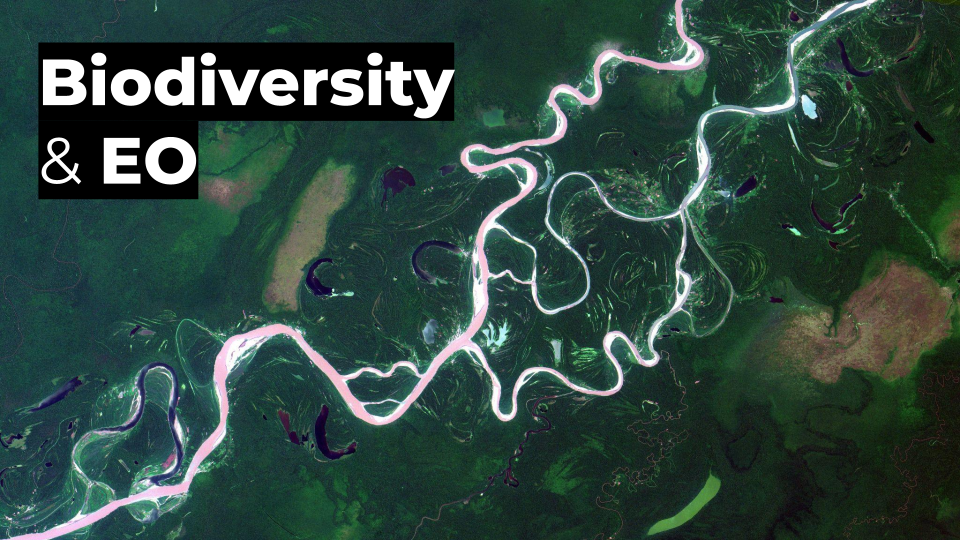
Applications of satellite technology in biodiversity conservation
Editor’s note: This article was written as part of EO Hub – a journalistic collaboration between UP42 and Geoawesome. Created for policymakers, decision-makers, geospatial experts and enthusiasts alike, EO Hub is a key resource for anyone trying to understand how Earth observation is transforming our world. Read more about EO Hub here.
Biodiversity refers to the variety of living organisms that inhabit our planet, including plants, animals, fungi, and microorganisms. This diversity not only enriches the natural world but also plays a crucial role in sustaining the ecological balance and providing vital ecosystem services. Unfortunately, human activities such as habitat destruction, climate change, and pollution have led to a significant loss of biodiversity in recent years. To address this pressing issue, scientists and conservationists have turned to satellite data as a valuable tool for monitoring and managing biodiversity. In this blog post, we will delve into what biodiversity is, why it matters, and how satellite data can help in conserving it.
Understanding biodiversity and its crisis
Have you ever wondered what would happen if biodiversity didn’t exist? How serious would the consequences be? The truth is that without biodiversity, the entire support system for human and animal life would collapse. The Millennium Ecosystem Assessment (MEA, 2005) indicated that the main ecosystem services related to human functioning are fresh water, food, raw materials, medicines, nutrient cycling, wastewater treatment, regulation of infectious diseases and climate, and recreation. Human access to biotic and abiotic elements of the natural environment minimizes threats to both physical and mental health. Consequently, we must realize that the ecosystems around us are essential to life on Earth. We can’t just remove a species. Everything carries greater or lesser consequences.
Recent decades have been characterized by an unprecedented rate of global change. Just think of the doubling of the human population over the past 50 years, going hand-in-hand with increased life expectancy, the quadrupling of the global economy, and a billion people lifted out of extreme poverty. This remarkable growth, however, has come at the expense of the natural environment, which has increasingly transformed and exploited for human needs and activities. This may be our last opportunity to save the biodiversity of our ecosystem, which is crucial to our future survival. At the Rio+20 Conference on Sustainable Development, UN member states reaffirmed “the seriousness of the global loss of biodiversity and ecosystem degradation, and highlighted the negative impact of this situation on food security, nutrition, access to water, the health of the rural poor and people around the world.”
The essence of data in supporting biodiversity
Achieving the UN Sustainable Development Goals and the Convention on Biological Diversity goals requires an evidence-based approach to conservation management. There is a need to analyze high-quality monitoring data to inform decision-making and evaluate policy interventions. In addition, the scientific community needs access to global, long-term, reliable information on spatio-temporal changes in the distribution of direct and indirect anthropogenic pressures on biodiversity. Information about the distribution, structure, composition and functioning of ecosystems is needed, as well as evidence of the effectiveness of various management actions. Equally important is the rapid detection of direct anthropogenic and natural threats to sensitive species and ecosystems. We are talking about habitat degradation – floods, deforestation, fires and illegal activities, such as poaching and hunting, among others.
Species distribution models (SDMs)
Species distribution models (SDMs) combine observations of species occurrence with integral biological attributes and environmental data (temperature, monthly precipitation, etc.). This is aimed at predicting the dynamics of species populations, i.e., their abundance over time and space, and the variability of geographic distribution. Changes in ecosystem functioning are largely influenced precisely by changes in species abundance and occurrence. SDMs have a rich range of applications. Among other things, they are used to model the historical distributions of species, predict the potential impact of climate change on their populations, and estimate the future growth of invasive species. It is one of the most important methods used in theoretical and applied ecological research. However, traditional SDM studies have usually relied on records of species occurrence from national atlases and herbariums, which has often generated many errors and inaccuracies. In addition, field surveys of habitats and populations are popular, but such a solution is extremely time-consuming. Thus, there is an emerging need for new and better data to speed up work and minimize the risk of error. Therefore, the opportunity for advances in ecological modeling lies in integrating the species distribution models with remote sensing technologies.
Application of EO data to remote sensing of environmental conditions
We can divide ecological factors into abiotic and biotic – they regulate the distribution and abundance of populations. Abiotic factors are non-living elements of the environment that directly or indirectly affect living organisms (water, terrain, climate, etc.). Satellite remote sensing offers continuous and regular observations of these climatic parameters. The first parameter we will look at is land surface temperature (LST), which measures thermal radiation emissions from land surfaces (bare soil, vegetation canopy, etc.) heated by incident solar energy. It is a key input indicator for climate models, as well as ecological or hydrological models. LST derived from remote data readings enables better SDM forecasting. Data products from Terra and Aqua MODIS and NPP VIIRS have been successfully integrated into SDM studies to improve simulations of ecological processes and biodiversity changes.
Estimating changes in precipitation over space and time is a key aspect of drought early warning and monitoring the impact of climate change. This is why controlling precipitation – another key environmental parameter – is so important in ecological modeling. Precipitation has a significant impact on the productivity of ecosystems and on the biodiversity of plant species and their distribution. NASA’s Precipitation Measurement Missions (PMMs) provide a continuous, long-term record of precipitation data through the Global Precipitation Measurement Mission (GPM) and the Tropical Rainfall Measurement Mission (TRMM).
Soil moisture is another important parameter for understanding ecosystem productivity. It controls the amount of water, replenishes aquifers, and can contribute to excessive runoff. It greatly influences the diversity of fungi and the distribution of plant species. NASA’s Soil Moisture Active Passive (SMAP) satellite is very useful here. It takes moisture measurements in the top 5 cm of soil around the world every 2-3 days with a spatial resolution of 9-36 km. Studies have shown that synthetic aperture radar (SAR) also has great potential to estimate surface moisture at higher spatial resolution.
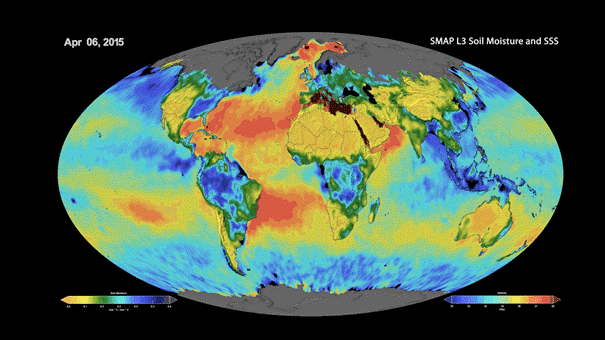
This animation shows a time lapse of sea surface salinity and soil moisture from NASA’s Soil Moisture Active Passive (SMAP) satellite from April 2015 through February 2019
Biotic factors, on the other hand, are the effects of organisms directly or indirectly on other organisms. Here remote sensing plays an important role in the remote detection of indicators of photosynthetic activity. In particular, the vegetation difference index (NDVI) is often used to predict the biodiversity of ecosystem plant species in different climatic areas. Additionally, the index can be used as a predictor of food availability for habitat modeling. MODIS NDVI products have been used in this way to model vervet habitats in Africa (you can read more about it here).
Remote sensing of species distribution
In SDM studies, data on presence, absence and abundance are extremely rare. Satellite and aerial remote sensing come to the aid, providing high-resolution images over large geographic areas. With this technology, it is possible to verify the spatial errors inherent in field surveys and, most importantly, save valuable time when surveying populations.
For remote plant detection, a condition must be fulfilled – the intended species must have a unique phenology or growth form. Only then will the use of surface reflection in the visible and near-infrared range yield results. Of course, it is often possible to distinguish vegetation types such as deserts, forests and swamps, but in order to analyze a particular species, its spectral characteristics must be distinguished. Hyperspectral remote sensing is indispensable here, combining spectroscopy and imaging. The acquired data sets usually consist of 100-200 spectral bands, greatly increasing the ability to distinguish the characteristic spectral signatures of individual plant species. In their paper, Tree species discrimination in tropical forests using airborne imaging spectroscopy, J. Féret and G.P. Asner successfully used HyMap, a hyperspectral scanner. It provides 128 bands in the reflective region of the solar wavelength 0.45 – 2.5 um with continuous coverage with an average bandwidth of 15 nm. This allows for highly accurate results in plant species detection studies.
However, NDVI can be useful not only for studying vegetation but also animals. As it turns out, changes in the distribution of NDVI over time can be correlated with the range of daily and seasonal movements of animal species. Another example of using space data for animal observation is the ICARUS (International Cooperation for Animal Research Using Space) Initiative. This is an international effort to develop a satellite-based observation system for small animals such as birds, bats and turtles. As part of the process, Icarus scientists will attach mini-transmitters to various animal species. These transmitters will then send their measurement data to the International Space Station (ISS). The receiving station will, in turn, transmit the data to the ground station, from where it will be sent to the relevant teams of researchers.
Conclusions
The opportunities for advancing conservation science through the development of innovative remote sensing products are promising. Using new technologies will allow faster and more accurate studies of biodiversity. Space agencies are constantly launching satellite remote sensing – the CEOS Missions, Instruments and Measurements (MIM) database currently contains detailed information on about 300 Earth observation satellite missions and 830 instruments currently in operation or scheduled to be launched in the next 15 years. However, remote sensing data products are not yet fully utilized and will contribute more to SDM for biodiversity monitoring and policy. It is becoming increasingly easy these days to obtain multispectral and optical data with very high resolution. Manyproviders offer data that are already corrected and analyzed, which can make the work much easier. Incorporating satellite remote sensing into the standard elements of an ecologist’s toolkit will certainly bring tangible benefits to biodiversity research.
Did you like the article? Read more and subscribe to our monthly newsletter!
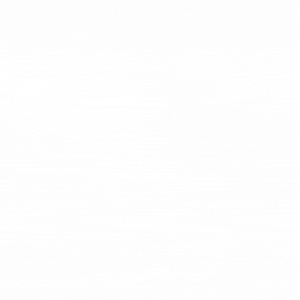